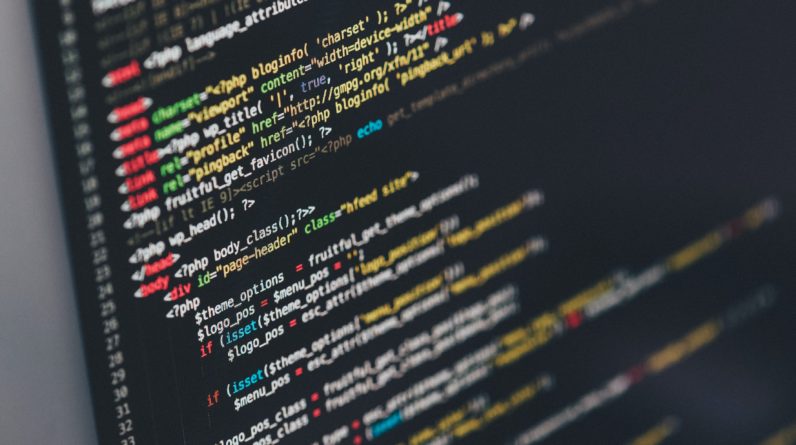
The Rise Of Quantum Computing In 2024
Imagine a world where complex computations are completed in seconds, where breakthroughs in medicine and climate modeling become the norm, and where the impossible suddenly becomes possible. Well, my friend, that world is not as far off as you might think. The rise of quantum computing in 2024 is upon us, and it brings with it a wave of implications and advances that will revolutionize the way we think, innovate, and solve problems. In this article, we will explore the exciting possibilities that lie ahead, uncovering the potential of this groundbreaking technology and how it will shape our future. Brace yourself for a journey into the future of computing that will leave you in awe.
The Basics of Quantum Computing
Introduction to quantum computing
The rise of Quantum computing is an emerging field with the potential to revolutionize the world of computing as we know it. Unlike classical computers that use bits to represent information as either a 0 or a 1, quantum computers use quantum bits or qubits, which can represent both 0 and 1 simultaneously thanks to the principles of quantum mechanics. This concept allows quantum computers to perform calculations at an exponentially faster rate, enabling them to solve complex problems that are beyond the capabilities of classical computers.
Key principles of quantum computing
Quantum computing is built upon several key principles of quantum mechanics. Two fundamental principles are superposition and entanglement. Superposition allows qubits to exist in a combination of 0 and 1 states simultaneously, exponentially increasing the computational possibilities. Entanglement, on the other hand, connects qubits in a way that the state of one qubit is dependent on the state of another, regardless of the distance between them. This phenomenon enables the creation of quantum gates and operations, resulting in powerful computations.
Quantum bits (qubits)
The fundamental building block of quantum computing is the qubit. Unlike classical bits that can be either 0 or 1, qubits can exist in a superposition of both states simultaneously. This property exponentially increases the computational power of quantum computers, as each additional qubit doubles the number of possible states. Qubits can be implemented through various physical systems, such as atoms, ions, photons, or superconducting circuits, each with its own advantages and challenges.
Quantum gates and operations
Quantum gates are the equivalent of logic gates in classical computing, but they operate at the quantum level. These gates manipulate the quantum state of qubits, allowing for complex computations. Common types of quantum gates include the Hadamard gate, which puts a qubit into superposition, and the CNOT gate, which creates entanglement between two qubits. By combining various quantum gates, complex algorithms can be executed on quantum computers.
Quantum parallelism and superposition
One of the most powerful concepts in quantum computing is quantum parallelism, which arises from the superposition property of qubits. Quantum parallelism allows multiple calculations to be performed simultaneously, significantly speeding up computations for certain algorithms. It enables quantum computers to explore multiple solutions to a problem in parallel, effectively providing exponential speedup over classical computers for certain types of problems.
Current State of Quantum Computing
Current limitations and challenges
Despite the tremendous potential of quantum computing, there are several current limitations and challenges that need to be addressed. One major challenge is the fragile nature of qubits, which are highly susceptible to disturbances from external factors such as noise and temperature fluctuations. This makes it difficult to maintain coherence and stability, limiting the computational capabilities of quantum computers. Additionally, the number of qubits currently available is still relatively small, making it challenging to solve complex real-world problems.
Overview of existing quantum computers
While still in its early stages, there are several notable quantum computers that have been developed and are being actively researched. Companies such as IBM, Google, and Microsoft have made significant progress in building quantum computers with increasing numbers of qubits. IBM’s Quantum Experience, for example, provides access to a cloud-based platform where users can execute quantum algorithms using real qubits. These existing quantum computers serve as important testbeds for further advancements in the field.
Comparison with classical computers
Quantum computers have the potential to outperform classical computers in certain problem domains. While classical computers excel at tasks that require sequential processing, quantum computers can leverage their parallel processing power to efficiently solve complex optimization and simulation problems, which are computationally expensive for classical computers. However, it’s important to note that quantum computers are not designed to replace classical computers but rather to complement them in solving specific problems.
Quantum supremacy and its implications
The rise of quantum computing is anchored in the pursuit of quantum supremacy, defined as the moment when a quantum computer can execute tasks beyond the reach of classical computers. This pivotal achievement in quantum computing would not only showcase the exceptional computational capabilities of quantum machines but also signal profound impacts in areas like cryptography and optimization. As a focal point of research, the race towards realizing quantum supremacy is propelling significant progress in the field of quantum computing..
Leading companies and research institutions
Several major companies and research institutions are at the forefront of quantum computing research and development. Companies such as IBM, Google, Microsoft, and Intel have made substantial investments in quantum computing and are actively working towards commercializing the technology. Additionally, research institutions and universities worldwide are conducting cutting-edge research in quantum theory, algorithms, and hardware development. The collaborative efforts between industry and academia are crucial in pushing the boundaries of quantum computing.
Applications of Quantum Computing
Quantum cryptography and secure communication
Quantum computing has the potential to revolutionize cryptography by enabling secure communication through unbreakable quantum encryption protocols. Quantum cryptography takes advantage of the principles of quantum mechanics, such as the no-cloning theorem and entanglement, to establish secure communication channels. By harnessing these quantum properties, quantum cryptography can provide a level of security that is theoretically resistant to hacking, ensuring the confidentiality and integrity of sensitive data.
Optimization and simulation problems
The rise of quantum computing has revolutionized the approach to optimization and simulation challenges common in sectors like logistics, finance, and material science. Traditional optimization methods typically falter when sifting through innumerable potential solutions. However, quantum algorithms, such as the Quantum Approximate Optimization Algorithm (QAOA), utilize quantum parallelism to navigate these possibilities more effectively. In parallel, quantum simulation methods are adept at modeling quantum systems, providing scientists with the tools to investigate intricate phenomena and innovate in material development.
Machine learning and AI
Quantum computing holds great promise for the field of machine learning and artificial intelligence (AI). Quantum machine learning algorithms have the potential to enhance and accelerate AI tasks by efficiently processing large datasets and optimizing complex models. Quantum-inspired algorithms, such as the Quantum Support Vector Machine (QSVM) and Quantum Neural Networks (QNN), are being developed to leverage the unique properties of quantum computing to improve the performance of AI systems.
Drug discovery and molecular modeling
Quantum computing can have a profound impact on drug discovery and molecular modeling. Simulating and understanding the behavior of molecules and complex chemical reactions is a computationally intensive task that can benefit from the quantum parallelism of quantum computers. With the ability to accurately model molecular interactions, quantum computers can revolutionize the discovery of new drugs and accelerate the development of personalized medicine.
Financial modeling and risk analysis
Quantum computing has the potential to transform financial modeling and risk analysis by providing faster and more accurate computations. Complex problems in portfolio optimization, risk assessment, and option pricing can be solved more efficiently using quantum algorithms. This can enable financial institutions to make better-informed decisions, manage risks more effectively, and optimize investment strategies. Quantum computing could play a crucial role in driving innovation and efficiency in the financial sector.
Advancements in Quantum Hardware
Scaling up the number of qubits
One of the key challenges in quantum computing is scaling up the number of qubits. Currently, the number of qubits available in quantum computers is limited, and increasing this number is essential to tackle more complex problems. Researchers are exploring different physical systems and qubit implementations, such as superconducting circuits, trapped ions, and topological qubits, to overcome the limitations of existing qubit technologies and scale up the number of qubits.
Improving qubit stability and coherence
Maintaining qubit stability and coherence is crucial for the reliable operation of quantum computers. Qubits are highly sensitive to environmental noise and disturbances, which can result in errors and loss of information. To improve qubit stability and coherence, researchers are exploring advanced error correction techniques, such as quantum error correction codes, and developing new materials and fabrication methods that minimize noise and decoherence.
Exploring different qubit implementations
There are various qubit implementations being explored in the quest for building scalable and error-tolerant quantum computers. Superconducting qubits, for example, offer promising scalability and have been used in some of the leading quantum computers. Trapped ion qubits, on the other hand, offer exceptional coherence but face challenges in scaling up the number of qubits. Other qubit implementations being researched include topological qubits and photon-based qubits. Each qubit implementation has its own advantages and challenges, and ongoing research is focused on optimizing their performance and stability.
Hybrid systems and error correction
Combining quantum and classical computing elements in hybrid systems can help address the challenges of scaling up quantum computers. By integrating error correction techniques and leveraging classical computing for error detection and correction, hybrid systems can enhance the stability and reliability of quantum computations. In addition, hybrid systems can leverage the strengths of both classical and quantum approaches, allowing for efficient and robust quantum computations.
Quantum network and interconnectivity
Building a quantum network that allows for the interconnection of multiple quantum computers is an active area of research. Quantum network protocols enable the transfer of qubits between different quantum processors, paving the way for distributed quantum computing and collaborative problem-solving. The development of a quantum internet could revolutionize fields such as secure communication, distributed computing, and quantum cloud services.
Quantum Algorithms and Programming
Development of quantum algorithms
Designing and developing quantum algorithms is a key focus of quantum computing research. Quantum algorithms exploit the unique properties of qubits and quantum gates to solve computational problems that are intractable for classical computers. Algorithms such as Shor’s algorithm for factorization and Grover’s algorithm for search demonstrate the potential power of quantum algorithms. Researchers continuously strive to discover new quantum algorithms and optimize existing ones to push the boundaries of what quantum computers can achieve.
Variational quantum algorithms
Variational quantum algorithms are a class of algorithms that combine classical and quantum computing elements. These algorithms leverage the iterative optimization of classical parameters to solve complex problems using the power of quantum computations. Variational quantum algorithms have shown promise in solving optimization problems, machine learning tasks, and molecular simulations. They offer a hybrid approach that allows for efficient utilization of both classical and quantum resources.
Quantum simulation techniques
Quantum simulation techniques aim to simulate physical systems using quantum computers. By leveraging the principles of quantum mechanics, quantum simulations can provide insights into complex quantum phenomena, such as the behavior of molecules or materials. Quantum simulators have the potential to revolutionize fields like chemistry, physics, and material science, enabling scientists to explore new frontiers of knowledge and develop innovative technologies.
Quantum error correction codes
Quantum error correction codes are essential for mitigating errors and enhancing the reliability of quantum computations. These codes encode quantum states in a redundant manner, allowing for the detection and correction of errors caused by external disturbances. Developing efficient and fault-tolerant quantum error correction codes is crucial for building large-scale, reliable quantum computers. Researchers are actively working on improving existing codes and exploring new coding techniques to address the fragility of quantum information.
Quantum programming languages and frameworks
As quantum computing evolves, there is a growing need for programming languages and frameworks that facilitate the development of quantum algorithms and enable efficient utilization of quantum resources. Several quantum programming languages, such as Q#, Qiskit, and Cirq, have been developed to abstract the complexities of quantum computing and provide high-level interfaces to quantum hardware. These programming languages are accompanied by frameworks that offer libraries and tools for quantum algorithm development, simulation, and execution.
Quantum Computing and Artificial Intelligence
Synergy between quantum computing and AI
Quantum computing and artificial intelligence (AI) have complementary strengths, and their convergence promises to drive significant advancements in both fields. Quantum computing can enhance AI by solving complex optimization and pattern recognition tasks more efficiently, while AI techniques can help filter and extract meaningful insights from vast amounts of data generated by quantum systems. The synergy between quantum computing and AI has the potential to revolutionize fields such as drug discovery, robotics, and cybersecurity.
Quantum machine learning algorithms
Quantum machine learning algorithms leverage the computational power of quantum computers to enhance classical machine learning tasks. These algorithms can process large datasets more efficiently using quantum parallelism and can optimize complex models through quantum-enhanced optimization techniques. Quantum machine learning algorithms have shown potential in improving tasks such as clustering, classification, and regression, paving the way for enhanced AI capabilities.
Quantum neural networks
Quantum neural networks are a variant of classical neural networks that leverage the principles of quantum mechanics to enhance their learning capabilities. By encoding data and weights into quantum states, quantum neural networks can process and model complex patterns more efficiently using quantum parallelism. These networks hold promise for addressing challenges in areas such as pattern recognition, image processing, and natural language processing.
Exploring quantum advantage in AI tasks
Researchers are actively exploring the potential advantage of quantum computing in various AI tasks. Quantum algorithms, such as the Quantum Support Vector Machine (QSVM) and Quantum Generative Adversarial Networks (QGANs), aim to outperform their classical counterparts by leveraging the inherent quantum properties of superposition and entanglement. While quantum advantage in AI tasks is still under exploration, early studies suggest that quantum computing can have a significant impact, especially for data analysis and pattern recognition problems.
Impacts on data analysis and pattern recognition
Quantum computing has the potential to significantly impact data analysis and pattern recognition tasks. With its ability to process massive datasets in parallel, quantum computing can accelerate data analysis tasks such as clustering, classification, and anomaly detection. Pattern recognition tasks, such as image and speech recognition, can also benefit from quantum algorithms that exploit the properties of quantum systems to efficiently extract and classify patterns. The combination of quantum and classical approaches can unlock new levels of insight and precision in data analysis and pattern recognition.
Quantum Computing in Industry and Research
Adoption of quantum computing by industries
Various industries are beginning to recognize the potential of quantum computing and are actively exploring its applications. For example, finance companies are exploring quantum algorithms for portfolio optimization and risk analysis, while healthcare institutions are investigating quantum simulations for drug discovery and personalized medicine. Energy companies are exploring quantum algorithms for optimizing power distribution and resource management. The adoption of quantum computing by industries is driven by the potential for significant advancements and breakthroughs in their respective fields.
Use cases in finance, healthcare, and energy
Finance, healthcare, and energy are three sectors that stand to benefit significantly from quantum computing. In finance, quantum algorithms can optimize investment portfolios, improve risk assessment, and enable real-time analysis of market data. In healthcare, quantum simulations can accelerate drug discovery and personalize treatment plans. Quantum algorithms can also optimize energy distribution, enable high-precision weather forecasting, and enhance resource management in the energy sector. These use cases showcase the potential for quantum computing to revolutionize critical industries.
Collaborations between academia and industry
Collaborations between academia and industry play a crucial role in advancing quantum computing research and driving its practical applications. Universities and research institutions contribute to the theoretical foundations of quantum computing and develop innovative algorithms and hardware technologies. Industry partners bring expertise in scaling up, commercialization, and the practical implementations of quantum technologies. These collaborations foster knowledge exchange, accelerate innovation, and bridge the gap between theoretical advancements and real-world applications.
Implications for scientific research
Quantum computing has the potential to revolutionize scientific research and enable breakthroughs in various disciplines. From unlocking the mysteries of quantum physics to simulating complex phenomena in chemistry and biology, quantum computers can augment scientists’ computational capabilities. Quantum simulations can be used to understand fundamental processes in nature, develop new materials with unique properties, and advance our understanding of the universe. The integration of quantum computing with scientific research opens up exciting possibilities for scientific discovery and innovation.
Ethical considerations and policy challenges
The rapid development of quantum computing presents several ethical considerations and policy challenges that need to be addressed. Quantum computing has the potential to break current encryption protocols, which raises concerns about data security and privacy in a post-quantum era. Policies and regulations need to be developed to ensure the responsible and ethical use of quantum computing. Additionally, the digital divide and access to quantum technologies need to be addressed to ensure equitable distribution of the benefits of quantum computing.
Emerging Quantum Technologies
Topological qubits and Majorana fermions
Topological qubits and Majorana fermions are emerging quantum technologies that hold great promise for building robust and error-tolerant quantum computers. Topological qubits leverage the exotic properties of topological materials to encode and protect quantum information, making them more resilient to errors caused by environmental disturbances. Majorana fermions, on the other hand, are particles that can be used as qubits and are the subject of intense research due to their potential for storing and manipulating quantum information.
Quantum annealing and adiabatic computation
Quantum annealing and adiabatic computation are alternative approaches to quantum computing that focus on solving optimization problems. Quantum annealing leverages quantum effects to find the lowest energy state of a given optimization problem. It has shown promise for solving combinatorial optimization problems and is being explored for applications in various fields. Adiabatic computation, on the other hand, exploits the adiabatic theorem of quantum mechanics to perform computations by gradually evolving the system to the desired solution.
Quantum sensors and metrology
Quantum sensors and metrology aim to leverage quantum phenomena to achieve unprecedented levels of precision and sensitivity in measurement devices. Quantum sensors exploit the quantum properties of particles to measure physical quantities, such as magnetic fields, electric fields, or temperature, with exceptional accuracy. Quantum metrology, on the other hand, focuses on improving the precision of measurements through quantum-enhanced techniques, such as entangled states and squeezed states. These emerging quantum technologies have applications in fields such as navigation, geophysics, and biomedical imaging.
Quantum interconnects and communication
Quantum interconnects and communication technologies are essential for building large-scale quantum networks and enabling secure quantum communication. Quantum interconnects provide physical connections between separate quantum processors, facilitating the transfer of quantum information. By establishing entanglement between distant qubits, quantum communication allows for secure and unbreakable transmission of information. These emerging quantum technologies are crucial for the development of a quantum internet and the realization of distributed quantum computing and secure quantum communication protocols.
Quantum machine learning hardware
Quantum machine learning hardware is an emerging field that aims to develop specialized quantum computing architectures for enhancing machine learning tasks. By designing quantum hardware specifically tailored for machine learning algorithms, researchers aim to improve the efficiency and performance of quantum machine learning systems. Quantum machine learning hardware explores novel approaches to encoding and manipulating data in quantum states, as well as optimizing the quantum circuits required for implementing machine learning algorithms. This emerging field holds promise for unlocking the full potential of quantum computing in the domain of machine learning.
Societal and Economic Impact of Quantum Computing
Advancements in cryptography and security
Quantum computing has the potential to revolutionize cryptography and security. While current cryptographic methods rely on the computational infeasibility of factoring large numbers, quantum computers could break these encryption schemes using Shor’s algorithm. Therefore, the development of post-quantum encryption algorithms, which are resistant to quantum attacks, is crucial to maintain data security in a quantum-powered world. Quantum cryptography, leveraging the principles of quantum mechanics, offers secure communication protocols that are resistant to eavesdropping and tampering.
Impact on privacy and data protection
The development of quantum computing raises concerns about privacy and data protection. With the ability to break current encryption standards, quantum computers could expose sensitive personal and corporate information. This requires the development of new encryption methods that are resistant to quantum attacks. Additionally, the increased computational power of quantum computers could enable the analysis of vast amounts of data, potentially compromising privacy and raising concerns about data protection. Policies and regulations need to be in place to address these privacy and data protection challenges.
Job market transformation and skill requirements
The rise of quantum computing will have a transformative impact on the job market and the skills needed to thrive in the quantum era. As quantum technologies advance, there will be an increasing demand for professionals with expertise in quantum theory, quantum algorithms, and quantum programming. Additionally, the integration of quantum computing with various industries will create new job opportunities, requiring a combination of domain knowledge and quantum skills. Governments, educational institutions, and businesses need to invest in quantum education and training programs to build a quantum-ready workforce.
Economic opportunities and investments
Quantum computing presents significant economic opportunities and has the potential to drive innovation, economic growth, and global competitiveness. The emergence of quantum technologies will create new markets and industries, leading to job creation and economic development. Companies that develop and commercialize quantum technologies stand to benefit from the growing demand for quantum computing solutions. Governments and private investors are recognizing the economic potential of quantum computing and are investing in research and development, infrastructure, and talent to foster quantum innovation and capitalize on the economic opportunities.
Potential risks and global competitiveness
The advancements in quantum computing bring about potential risks and challenges for global competitiveness. Countries that lead in quantum research and development could gain a significant competitive advantage, both economically and strategically. The development of quantum technologies may lead to a redistribution of global power and influence, impacting industries, national security, and geopolitical dynamics. Ensuring a level playing field and fostering international collaboration are crucial to address these risks and secure a sustainable and equitable future in the quantum era.
Future Outlook for Quantum Computing
Predictions for the next decade
In the next decade, quantum computing is expected to witness significant advancements and breakthroughs. Researchers aim to build larger and more stable quantum computers, pushing the limits of qubit scalability and coherence. Quantum algorithms will continue to evolve, enabling the solution of increasingly complex problems and demonstrating quantum advantage in more domains. Additionally, the development of practical quantum error correction codes and fault-tolerant quantum computing will pave the way for the realization of large-scale, reliable quantum computers.
Path towards fault-tolerant and scalable quantum computers
The rise of quantum computing is contingent on surmounting key obstacles in creating fault-tolerant and scalable quantum computers. These challenges include enhancing qubit stability, minimizing errors, and shielding against external interferences. Researchers are delving into diverse qubit models, pioneering error correction methods, and developing innovative quantum architectures to tackle these issues. Employing classical error correction strategies alongside hybrid methodologies will bolster the reliability of quantum computations and aid in the expansion of quantum computer capabilities. It’s through these technological strides that the true capacity of quantum computing will be unleashed.
Opportunities for interdisciplinary research
Quantum computing presents vast opportunities for interdisciplinary research, bringing together experts from various fields to tackle complex problems. Collaboration between quantum physicists, computer scientists, mathematicians, engineers, and domain experts in industries such as chemistry, finance, and healthcare will drive innovation and pave the way for transformative applications. By combining expertise and perspectives from different disciplines, interdisciplinary research can unlock new possibilities and foster a deeper understanding of the potential of quantum computing in solving real-world problems.
Exploration of quantum networking and quantum internet
The exploration of quantum networking and the development of a quantum internet are key focuses in the future of quantum computing. Quantum networking aims to establish connections between quantum processors, enabling distributed quantum computing and secure quantum communication. The development of a quantum internet would revolutionize fields such as cryptography, secure data transfer, and cloud services. Researchers are working towards building quantum network protocols, quantum routers, and quantum repeaters to enable the seamless transfer of quantum information over long distances.
Cultural, political, and educational implications
The rise of quantum computing will have cultural, political, and educational implications that need to be considered. Quantum technologies could reshape industries, economies, and societies, influencing cultural norms and social dynamics. Political considerations, such as national security and strategic interests, will shape policies and regulations around quantum research and development. Educational institutions need to adapt to prepare future generations for the quantum era, offering specialized curricula and programs that foster quantum literacy and technological fluency. The cultural, political, and educational implications of quantum computing will play a pivotal role in shaping the future of this transformative technology.